1. Introduction
Upper limb wearable devices are designed to be used or implanted on the arms, hands, or fingers. These devices can be used for various purposes, such as rehabilitation of people with movement disorders, as assistance with daily activities for people with physical disabilities, and as prosthetic devices for people who have lost the use of their limbs. These devices may use a combination of sensors, actuators, and control algorithms to assist or replace the function of the upper limb. These devices can be an external structural mechanism with joints and links corresponding to those of the human body (Rosen & Perry, 2007) or, more recently, be portable and flexible soft exoskeletons to assist the flexion of the upper limbs (Samper-Escudero et al., 2020b). Some research papers present review studies that go through particular aspects regarding upper limb devices, namely upper limb prostheses and rehabilitation technology (e.g., Bardi et al., 2022; Biddiss & Chau, 2007; Cordella et al., 2016; Lo & Xie, 2012; Walmsley et al., 2018; Wang et al., 2017).
Biddiss and Chau (2007) present a review of the acceptance and abandonment of upper limb prostheses from 1980 to the beginning of 2006, identifying the factors associated with abandonment and consumer dissatisfaction. Understanding and recognizing these factors is crucial for enhancing and refining the design of new devices, which is essential for optimizing the overall performance of these devices. They also highlight the significance of prioritizing participatory research and consumer satisfaction, which is crucial to ensure that the design of devices effectively closes the gap between the research lab and the clinic and between the clinic and the home.
Lo and Xie (2012) review and discuss using upper limb exoskeleton robots to rehabilitate patients with neuromuscular disorders, mainly to provide optimal rehabilitation therapy for stroke victims. Cordella et al. (2016) follow similar concerns regarding the limitations of current prosthetic solutions for people with limited autonomy and ability to perform daily living, working, and social activities. They propose a list of requirements for upper limb prostheses based on user needs to improve user satisfaction and reduce device abandonment. Walmsley et al. (2018) present and discuss the use of wearable sensors to calculate upper limb joint angle and their potential as viable instruments for measurement during active movement. Wang et al. (2017) listed and classified interactive wearable systems for movement and posture monitoring during upper body rehabilitation, regarding the sensing technology, system measurements, and feedback conditions and evaluating their wearability and clinical effectiveness. By doing this, they demonstrated that this area of research engages different backgrounds, not only from rehabilitation and biomedical sciences but also from computer science and engineering.
Burton (2020) discusses the various uses for exoskeletons and exosuits, including for rehabilitative care, industrial manufacturing, leisure activities, and military combat, highlighting that exoskeletons can be powered, passive, pseudo-passive, or active, and may be soft or rigid materials. The author also states that the substantial technological overlap between different uses allows for easy adaptation of exoskeletons and exosuits for various purposes. The ABLE 7-axes upper limb exoskeleton is an example of repurposing from stroke rehabilitation to industrial manufacturing. Exoskeletons can also be designed to reduce arm weight when carrying tools such as screw guns or paint guns. Elstub et al. (2021) define exoskeletons and exosuits (exos) as wearable devices that enhance physical activity, posture, and motion; devices that biomechanically assist with a variety of tasks, including bending, lifting, reaching, walking, running, and jumping, and are used in various applications, including clinical and occupational settings.
Pérez Vidal et al. (2021) discuss the characteristics and functionality of soft exoskeletons, analyzing investigations conducted over the past decade on upper and lower joints, including the shoulder, elbow, wrist, hand, hip, knee, and ankle. The authors examine factors such as degrees of freedom, force, actuators, power transmission methods, control systems, and sensors. Exoskeletons are designed to aid the movement of various joints in the body, with single or multiple degrees of freedom, depending on the complexity and physical capabilities of the device. The authors also classify exoskeletons based on their actuation devices, which can be electric, pneumatic, hydraulic, or hybrid.
More recently, Bardi et al. (2022) reviewed the current approaches in designing and producing soft robotic wearable devices, specifically upper limb exosuits, grouped according to actuation type. The authors conclude that while few devices are ready for market, exosuits show high potential for daily activity assistance, and clinical trials with shared evaluation metrics are needed to assess their effectiveness. Exosuits are a recent valid alternative for the disadvantages of exoskeletal structure devices in restoring human physical abilities and improving performance and quality of life. Exosuits, identified as soft robotic devices, are typically designed to be worn over the user's limbs, torso, or entire body and can provide not only assistance with activities of daily living and rehabilitation but also be used in military and industrial applications (Samper-Escudero et al., 2020b).
Indeed, upper limb wearable devices have a wide range of applications. However, most research seems to focus on medical applications, including rehabilitation, assistance with daily activities, and prosthetic devices. Although numerous reviews discuss these systems, none establish the linkage between concept clusters or trace their evolution over time. There is an intricate lexicon that may bring confusion regarding the significance of terms that may seem synonyms. Hence, the primary objective of the present study is to gain insights into the evolution of these devices in correlation with other concepts, thereby enhancing knowledge regarding their application trends and emerging areas within the field.
This research endeavors to shed light on the evolution of the technology, as mentioned earlier, through a systematic literature review (SLR) by posing two research questions (RQ):
RQ1: “How has this technology been evolving?”, and
RQ2: “What is the trend according to the fields of application?”.
RQ1 seeks to understand the trajectory of this technology, exploring its advancements and changes over time. By examining its evolution, the study aims to uncover the key developments, breakthroughs, and transformations that have shaped its current state.
RQ2 aims to investigate the trend of this technology within various fields of application. By analyzing its utilization across different domains, the study intends to identify emerging areas where the technology has gained prominence and ascertain the extent to which it has been adopted.
2. Methods
A Systematic literature review (SLR) was conducted, followed by descriptive statistical analysis. The free software VOSviewer (van Eck & Waltman, 2023) was used to construct and view bibliometric maps. As a research method, SLR includes comprehensive literature searches, well-defined inclusion and exclusion criteria, systematic quality assessment, and careful synthesis and interpretation of findings (Silva et al., 2022; Thomé et al., 2016). VOSviewer was selected because of its user-friendly graphical interface and ability to generate maps of co-authorship and co-citation networks, which are useful inputs for the analysis. The findings allowed for reducing the number of documents to 26 considered for the critical qualitative synthesis. Combining the information gathered enabled addressing the extent and emerging trends in the development of upper limb embedded devices according to the field in which they are applied.
2.1 Search Methods
The SLR was conducted based on all published studies on upper-limbed embedded devices and their application areas considering the Scopus database. The combination of keywords:
[upper-limb* AND wearable* AND device*]
was used in the title, abstract, and keywords fields. The character "*" allowed finding words with the same stem, that is, all possible characters after the last letter (e.g., plural of each word). Since the search is not case-sensitive, the hyphen is considered a space. So, the use of "upper-limb" is equal to the use of "upper limb”.
No restriction to the time frame was considered allowing us to verify the evolution of this topic over time and whenever a change is made. Only articles, conference papers, and book chapters were considered. The search, conducted in December 2022, yielded 555 documents. This number was obtained after excluding not available full documents.
2.2 Data collection and analysis
Considering all 555 documents, a descriptive analysis was performed, which facilitated a comprehensive understanding of the overall trends and patterns. The analysis helped refine and select the data results to answer this study's main research questions.
After conducting a search of the Scopus database, it was found that the earliest publications on this topic appeared in 2002. Beginning in 2015, a clear trend of a rapid increase in the number of documents published, which is almost exponential in nature. However, with the onset of the pandemic in 2020-2021, there was a noticeable decrease in the number of publications. As seen in Fig. 1, there has been a slight increase in the number of publications in 2022.
The majority of the publications (57%) were in the form of articles, followed by conference papers (39%) and book chapters (4%). In terms of subject areas, the majority of the publications (72.5%) fell into four major categories, with engineering being the most significant (29%), followed by computer sciences (22%), medicine (15%), and biochemistry, genetics, and molecular biology (6.5%). These findings are presented graphically in Fig. 2.
Sixty countries were identified with at least one document. Table 1 lists the top 12 countries with their documents' frequencies in descending order of the total link strengths. The United States of America (USA) had the highest number of publications, with 115 (20.7%), and the highest total link strength value. Italy had the second-highest number of publications, with 90 (16.2%), followed by China with 74 (13.3%).
The UK and Japan had 45 publications each (8.1%), with the UK having a higher total link strength value (25) than Japan's 17. The total link strength is a measure of the overall strength of the citation links between a particular document and other documents.
Fig. 3 displays the clusters defined in which each country is represented by a sphere whose size is proportional to the number of documents, and the color indicates the average number of citations.
Fig. 4 displays the average publication year for documents published between 2015 and 2022. Notably, the number of documents published on this topic has been steadily increasing over the years, and it is interesting to observe that European countries such as Germany and France have recently begun to publish research related to this topic, as indicated by the yellow-colored spheres in Fig. 4.
3. Results and Discussion
The results described in the previous section will help refine and select the data results to answer the research question RQ2, "What is the trend according to the fields of application?”. Thus, two steps were taken. Firstly, a continuation of the descriptive analysis of the 555 documents was conducted, which included a citation analysis of relevant keywords. Secondly, the analysis was refined by identifying the most representative clusters of keywords, and then, the data were organized to identify different themes and the relationships between them.
3.1 Keyword Citation Analysis
A co-occurrence analysis was performed considering the keywords (indexed and author). A keyword is not only a word or phrase that summarizes the main topic of a document but also assists in classifying the information and streamlining the process of searching and retrieving information from a database or search engine. Therefore, analyzing the co-occurrence of keywords can help identify trends, which can be helpful for further development in a research domain.
Fig. 5 depicts the network that was generated from the bibliographic data for the keyword co-occurrence map. In the visualization, the distance between two keywords approximately indicates their relatedness in terms of co-occurrence links. Thus, the closer two keywords are located to each other, the stronger their relatedness is. This network was constructed based on 1316 keywords, subsequently reduced to 63 by defining a minimum occurrence threshold of 5 for each keyword. The seven clusters obtained are distinguishable by their colors: red for "wearable sensors", green for "wearable device", "exoskeletons", and "human-robot interaction", blue for "stroke" and "activities of daily living", yellow for "rehabilitation robotics" and "soft robotics", purple for "rehabilitation", light blue for "assistive devices" and "haptics", and orange for "exoskeleton" and "wearable robot". Notably, "exoskeletons" also appear in the green cluster alongside "exosuit" and "upper limbs".
Furthermore, an analysis of the keywords by year was conducted, indicating that the adoption of the most recent keywords ("Exoskeletons", "exosuit", and "upper-limbs") occurred after 2020, as shown in Fig. 6. This figure was created based on the average occurrence score of the keywords per publication year.
Examining Fig. 6 from left to right, one can observe the evolution in using keywords related to upper-limb embedded devices. In 2018, the keywords were primarily linked to "exoskeleton" and "activities of daily living", followed by "wearable device" and "soft robotics". Subsequently, around 2020-2021, new developments emerged, highlighting new areas of applications based on "exosuit" and the incorporation of machine learning. Machine Learning is used to interpret the sensor's signal in a personalized way according to the user.
Exoskeletons and exosuits are wearable devices that can enhance human capabilities, but they are designed for different purposes and have different features (Burton, 2020; Elstub et al., 2021; Pérez Vidal et al., 2021). As previously mentioned, exosuits, also known as exoskeleton suits, are more lightweight and flexible than exoskeletons and can also be used by healthy people to enhance their performance by assisting with industrial applications, as illustrated in Fig. 7 or daily living or surgical or precision tasks when performed under extreme ambient temperature conditions, among others (Fu et al., 2022). These two types of technological devices, exoskeletons, and exosuits, are still in the early stages of development, and technology is evolving rapidly.
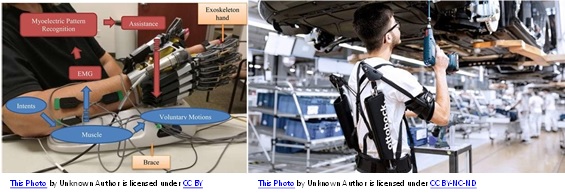
Figure 7 Examples of two types of Exoskeletons and exosuits devices that enhance human capabilities.
Combining the information gathered makes it possible to comprehend the driving force behind upper limb embedded systems and grasp how it has progressed to increasingly optimized systems, allowing to follow the second step refining the analysis considering the green cluster of keywords (see Fig. 5).
3.2 New Trends Analysis
As previously referred to, "exosuits" is a keyword that started to be used recently. So, of the 555 documents initially identified, 26 used "exosuits" as a keyword to summarize the main topic of the work developed, which were then selected for further critical analysis. The authors reviewed all papers individually and then together to compare and discuss their initial findings. Any disagreements were resolved through discussion and reached a shared consensus. During this process, the data were iteratively analyzed, identifying patterns or categories and classifying them into meaningful descriptions or codes, generating new insights, meaning, and significance of the data according to the objectives. The final codes yielded were: target population, activity, type of final product, and main results obtained. This set of codes was selected to facilitate the analysis to address and answer the research question RQ2 effectively. So, to identify the field of application, the codes target population, activity, and type of final product, which naturally seemed to be the most appropriate and easy to identify through the reading of the selected documents. The fourth code, the main results obtained, was also considered because it allows the identification of the final result of the scientific work described, reinforcing the current state of technological and scientific development of this topic. The authors’ scientific backgrounds collectively contributed to the accomplishment of this stage, with each author's unique background and years of experience adding valuable perspectives and insights to the discussion. To emphasize knowledge and know-how in human factors in engineering and technology development in electronic and computer engineering.
Table 2 lists, by descending order of publication date, and summarizes the primary descriptions of these 26 documents according to the four considered codes.
Of the 26 articles analyzed, most describe the device (18) to be used in the rehabilitation of patients who have suffered some accident (impairments muscular dystrophies, by stoke) that made it impossible to use the upper limbs (approximately 6) and in everyday tasks, the rest to be used in an occupational environment as support and extension to manual tasks (2). Some articles do not explicitly refer to the population to which the device is suitable, referring to a general application in its application: in rehabilitation, either to help in the execution of tasks that require effort or to help in the tasks and movements of astronauts. The remaining 12 refer to the development of the models/algorithms and mechanisms that are attached to the device, the control platform, and the sensors needed to follow the movements of the upper limbs.
It is noteworthy that the identification of two teams that develop this type of device is visible. On the one hand, the Samper-Escudero team, with the description of the developments of LUXBIT, a cable-driven flexible exoskeleton that combines textiles and sewing patterns to anatomically adapt and promote freedom of movement in outdoor and workspace applications. On the other hand, Lessard's team with CRUX, portable equipment with a mimetic control algorithm and mechanical functionality, offers a lightweight, compliant upper-extremity solution to augment human movements while preserving flexibility.
4. Final Considerations
The systematic literature review (SLR) provides a comprehensive understanding of exoskeletons and exosuits, their differences, and how they function. Exoskeletons and exosuits are wearable devices project8ed to augment human capabilities in various applications. However, they differ in construction and weight: exoskeletons are often heavier and bulkier and started being developed for medical purposes to support and assist individuals with physical impairments and in industrial settings to reduce the risk of injury and fatigue among workers; exosuits are lighter and more flexible. Both exoskeletons and exosuits can also assist healthy individuals in enhancing their performance and reducing the risk of injury in sports and military settings. They have shown potential therapeutic benefits in rehabilitation and physical therapy by promoting muscle activation and improving gait patterns in individuals with mobility impairments. The versatility of exosuits makes them a promising area of research and development in wearable technology. industries, including manufacturing, healthcare, sports, and even in more disruptive activities, such as space exploration. Exoskeletons have been shown to augment their users' skills and physical abilities significantly. This technology has the potential to be particularly useful for astronauts during spacewalks, where the effects of microgravity can result in a significant loss of physical capabilities. In such scenarios, exoskeletons can provide the necessary support and assistance to allow astronauts to perform tasks more efficiently and precisely. Additionally, exoskeletons equipped with sensors and other advanced technologies can help monitor and regulate astronauts' vital signs and other health parameters, ensuring their safety and well-being during long space missions.
Although the analysis provides insights into the evolution and trends of exoskeletons and exosuits, as an SLR relies on existing literature, it may have inherent constraints and biases. It may not capture all aspects or address every research question comprehensively. The present work focused on the last decade, achieved through one selected database (Scopus). Documents without online publication versions available were not included. In further analysis, other databases, such as the Web of Science, could also be considered for broader searching. The search could be extended beyond the use of keywords by considering the iterative snowball backward and forward searches, which could help identify relevant literature that may have been missed and enhance the comprehensiveness of the SLR (Webster & Watson, 2002). Nevertheless, the findings offer a broad understanding of the development, differences, and applications of exoskeletons and exosuits, highlighting the importance of considering the human element in designing and implementing wearable technology. In short, by providing additional support, reducing fatigue and injury risk, and enhancing physical performance, exoskeletons and exosuits can improve human workers' quality of work and life. This progress aligns with the values of Industry 5.0, which seeks to optimize the relationship between technology and humans to benefit both. As a result, it is possible to observe an increasing focus on the development of exoskeletons and exosuits in recent years as the importance of the human element in manufacturing and other industries continues to be recognized.
The breakpoint observed in 2015 may also be an indicator of this transition between I4.0 and I5.0, as this year can be considered approximately the mid-term of the implementation of I4.0 and I5.0 (Sarfraz et al., 2021). As such, the next step in this research, among other things, involves considering the data divided into these two clusters (before and after I5.0 onset) regarding the publishing date, using 2015 as a breakpoint to understand better what may have caused the exponential trend afterward. A further step in this research would be to understand which areas have been tackled by some specific countries.